An Efficient Friendly Process for Synthesis of (2E,6E)-2,6-bis(4-nitrobenzylidene)cyclohexanone a Drug Against Dormant Version of the Mycobacterium Tuberculo-sis H37Ra
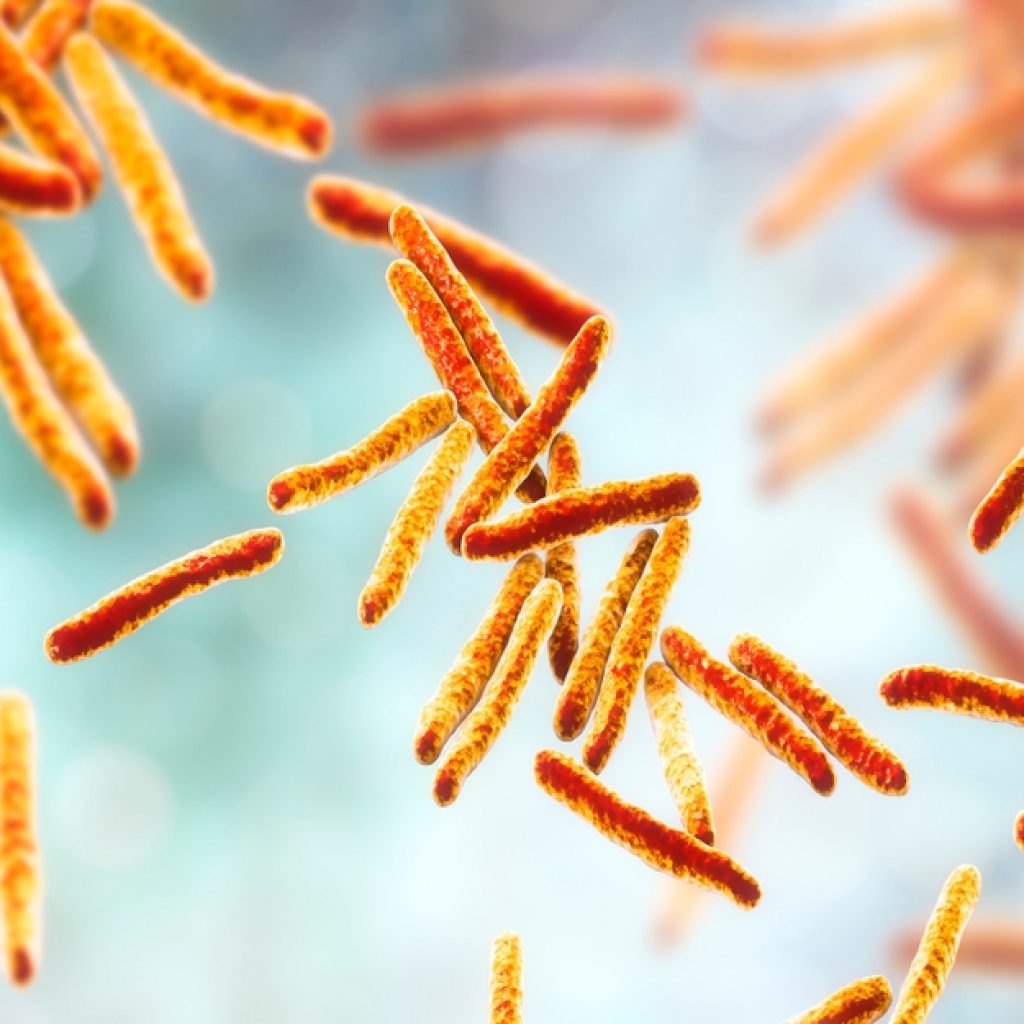
Prof.Dr Kannan Vishwanatth
The Chinese University of Hong Kong.
Emil: drkannanvish@yahoo.com
Dr Kannan Vishwanatth . An efficient friendly process for Synthesis of (2E,6E)-2,6-bis(4-nitrobenzylidene)cyclohexanone a drug against dormant version of the Mycobacterium tuberculosis H37Ra
Abstract: The objective of this study is the Synthesis of (2E,6E)-2,6-bis(4-nitrobenzylidene)cyclohexanone a drug against dormant version of the Mycobacterium tuberculosis H37Ra. The Cross-aldol condensation between 4-nitrobenzaldehyde and cyclopentanone catalyzed by modified fluorapatite Na/FAP catalyst in aqueous media is an important protocol for the synthesis of this product and the reaction conditions were optimized by the response surface methodology. The work-up procedure is simplified by simple filtration with high yield and by-products of usual undesirable reaction are not observed.
Keywords: (2E,6E)-2,6-bis(4-nitrobenzylidene)cyclohexanone, Anti-Tuberculosis Drug, Mycobacterium tuberculosis H37Ra, Modified Fluorapatite, Cross-aldol condensation
- Introduction
Tuberculosis (TB) remains a significant health problem with high morbidity and mortality [1]. The main cause of TB is Mycobacterium tuberculosis [2]. According to the World Health Organization (WHO), in 2014, 9.6 million people fell ill with TB and 1.5 million died from the disease. Over 95% of TB deaths occur in low- and middle-income countries, and it is among the top 5 causes of death for women aged 15 to 44. An estimated one million children (0-14 years) fell ill with TB, and 140 000 children died from the disease in 2014 [3].
However, about one-third of the world’s population do not develop the disease immediately upon infection with the Mycobacterium tuberculosis. Instead, the bug remains dormant in the body, enclosed in a capsule, which the host organism creates to protect itself. It may remain in this state for years, or even decades, before becoming active and triggering the development of the disease. This often happens when poor nutrition or a disease of the immune system weakens the host’s immune system [4].
The scientists from the Max Planck Society in Germany and Yonsei University in South Korea investigated the dormant state of the Mycobacterium tuberculosis and the mechanisms, which cause it to wake up and become active again [5]. They focused their efforts on two variants of the tuberculosis disease that differ in one crucial aspect; while strain H37Rv causes the development of the disease, strain H37Ra is harmless, remaining dormant in the host’s cells. These scientists discovered that the different behavior of the two strains is caused by minute genetic differences and that these differences could be traced back to a single mutation in a gene which codes for the protein PhoP in the harmless H37Ra strain. An intact PhoP is therefore clearly necessary for the bacteria to be able to cause tuberculosis. PhoP is a transcription factor, which binds to the DNA and so controls the activity of other genes. The mutated version of the gene lacked the ability to bind to the DNA correctly. These studies led to the so-called dormancy regulon, a group of genes that creates the proteins responsible for initiating and maintaining the dormant state. In the harmless H37Ra strain, these genes were far more active than in the virulent H37Rv strain. Probably the intact PhoP suppresses the genes of the dormancy regulon and so causes the bacteria to wake up. A defective version of the protein makes the bacteria less dangerous because it prevents it from waking up.
In any case, we urgently need medicines, which attack the dormant bacteria. Only in this way can we significantly reduce the unusually long treatment time of six months. A more precise understanding of the survival strategies of the tuberculosis bacteria offer the first starting point for the development of new medicines, which are more urgently needed than ever, as antibiotic-resistant tuberculosis bacteria are becoming more and more widespread [6].
In this context, the Indian authors [7] are showed that the (2E,6E)-2,6-bis(4-nitrobenzylidene)cyclohexanone presented an activity against Mycobacterium tuberculosis H37Ra. They found that sensitivity testing of the product by the minimum inhibitory concentration method (MIC) indicate a value of 3.12 μg/mL.
Cross-aldol condensation [8] is an important protocol for the synthesis of various bis-(arylmethylidene)cycloalkanones. This reaction is classically carried out using strong acid or base [9]. Indeed, different organometallic complexes, Lewis acid are found to be able to catalyze this reaction [10]. However, most of the above-mentioned process generated a large amount of toxic waste and involved tedious separation procedures since the reactions were homogeneous and the catalysts were destroyed or discarded on the work-up procedure [11].
Recently, because of increased environmental concerns and strong economic driver in chemical research and industry, great efforts have been made on heterogeneous catalysis, which allows the catalyst for easy isolation and recycling. Thus, solid catalysts have drawn considerable attention in organic synthesis due to their advantages such as environmental compatibility, reusability, high selectivity, simple operation, non-corrosiveness and low cost [12].
In spite of global research efforts, we describe in this paper, an efficient friendly process for modeling and synthesis of (2E,6E)-2,6-bis(4-nitrobenzylidene)cyclohexanone a drug against dormant version of the Mycobacterium tuberculosis H37Ra using Response Surface Methodology (RSM).
- Materials and Methods
2.1. Preparation and characterization of catalysts
The fluorapatite (FAP) have been prepared by reaction between diammonium phosphate, calcium nitrate and ammonium fluoride in presence of ammonia [12]. The FAP obtained was calcined at 900°C before use. The structure of this catalyst was confirmed by X-ray diffraction, infrared spectra and chemical analysis. The surface area of calcined FAP was determined by the BET method and found to be S=15.4 m2 g−1. The total pore volume was calculated by the BJH method (VT=0.058 cm3 g−1).
The modified fluorapatite (Na/FAP) has been prepared by impregnation of the FAP with a solution of sodium nitrate followed by calcination at 900°C [13]. The surface area of the new catalyst Na/FAP was determined by the BET method as 5.4 m2 g−1 and the total pore volume obtained by the BJH method is 0.0032 cm3 g−1.
2.2. General procedure
The general procedure for synthesis of (2E,6E)-2,6-bis(4-nitrobenzylidene)cyclohexanone is reported in Figure 1, as follows: To a flask containing a mixture by taking 2:1 mole ratios of 4-nitrobenzaldehyde 1 and cyclopentanone 2 in aqueous media, the Na/FAP catalyst was added and the mixture was stirred at reflux. Hot water (2×20 mL) was added, followed by simple filtration.
Figure 1. Synthesis of (2E,6E)-2,6-bis(4-nitrobenzylidene)cyclohexanone.
Hot After filtration and extraction with hot water, the solution was concentrated and purified by silica gel chromatography. Na/FAP was reactivated by drying at 150 °C or, alternatively washed with acetone and calcined at 600 °C for 1 h. The crude product 3 was identified by melting point, IR, 1HNMR, 13C NMR and fluorescence emission spectrum. The spectral analysis of this product is shown in Figure 2.
2.3. Statistical analysis
The synthesis of anti-tuberculosis drug (2E,6E)-2,6-bis(4-nitrobenzylidene)cyclohexanone by Cross-aldol condensation between 4-nitrobenzaldehyde and cyclopentanone catalyzed using Na/FAP in aqueous media was carried out and optimized using the Central Composite Design (CCD) and Response Surface Methodology [15].
CCD helps in investigating linear, quadratic, cubic and cross-product effects of the three reaction condition variables on the reaction yield. The two independent variables studied were reaction time (X1) and catalyst weight (X2). Table 1 lists the range and levels of the four independent variables studied.
Table 1. Study field and coded factors
Natural variable | Unit | Coded variables X1 and X2 | ||
-1 | 0 | +1 | ||
Reaction time | h | 3 | 4 | 5 |
Catalyst weight | mg | 200 | 400 | 600 |
Figure 2. Spectral analysis of (2E,6E)-2,6-bis(4-nitrobenzylidene)cyclohexanone.
The experimental data obtained by following the above procedure were analyzed by the response surface methodology using the following second-order polynomial equation 1:
Y = β0 +β1X1 +β2X2 (1)
+β11X12 +β22X22 +β12X1X2
Where Y is the response (reaction yield); Xi and Xj are the coded independent variables and β0, βi, βii and βij are intercept, linear, quadratic and interaction constant coefficients, respectively.
The software STATGRAPHICS-Plus was used for regression analysis and analysis of variance (ANOVA). The Response surfaces and the contour plots were developed using the fitted quadratic polynomial equation obtained from regression analysis, holding two of the independent variables at a constant value corresponding to the stationary point and changing the other two variables.
Confirmatory experiment was carried out to validate the equation, using combinations of independent variables which were not part of the original experimental design but within the experimental region.
- 3. Results and Discussion
By using a central composite design (CCD) a correlation between the reaction condition variables to the reaction yield was developed. Table 2 shows the complete design matrix and reaction yield at various reaction condition variables. Selection of the levels was based on our preliminary study. The value of α for this CCD was fixed at ±1.41421.
Table 2. Experimental design and results
Order | Coded units of variable | Reaction yield | |
X1 | X2 | ||
01 | +1 | +1 | 96 |
02 | +1 | -1 | 53 |
03 | -1 | +1 | 73 |
04 | -1 | -1 | 33 |
05 | +1.41421 | 0 | 85 |
06 | -1.41421 | 0 | 54 |
07 | 0 | +1.4142 | 87 |
08 | 0 | -1.4142 | 28 |
09 | 0 | 0 | 77 |
10 | 0 | 0 | 76 |
The complete design matrix of the experiments conducted and results are given in Table 2. All variables at zero level constitute to the center points and the combination of each of the variables at either it’s lowest (- 1.41421) level or highest (+ 1.41421) level with the order variables at zero level constitute the axial points. The experiment sequence was randomized in order to minimize the effects of the uncontrolled factors.
In table 2 we can show, the reaction yield obtained was in the range from 28 to 96%. Experimental error was determined from run 9 to 10 at the center point of the design.
A regression analysis was performed to fit the response function and predict the outcome of reaction yield with a simple equation. The model is expressed by equation 2 which takes their coded value.
Y = 76.5 + 10.8551*X1 + 20.8049*X2
– 3.4375*X12 – 9.4375*X22 + 0.75*X1X2 (2)
The summary of the analysis of variance (ANOVA) result is shown in Table 3. The regressors or term incorporated in the model are those statistically tested to be significant. The ‘Prob > F′ value indicates the probability equals the proportion of the area under the curve of the F-distribution that lies beyond the observed F value.
The small probability values called for the rejection of the null hypothesis, in other words, the particular term significantly affected the measured response of the system.
In this case, the linear terms (X1 and X2), the squared terms (X12 and X22) and the interaction term (X1X2) have P-values less than 0,05, indicating that they are significantly different from zero at the 95,0% confidence level. On the other hand, from this ANOVA the model F-value of 5225.16 implied that the model was significant. Values of Prob.> F0.01(5, 4)=15.52 less than 0.01 indicated that the model terms was significant. The coefficient of determination, R2 for the model was 99.98%. This indicates that only 0.02% of the total variability was not explained by the regressors in this model. The high R2 value specifies that the model obtained will be able to give a convincingly good estimate of response of the system in the range studied.
The influence of each independent variable on the response is shown in Figure 3. By looking at this figure, we see that the reaction time and the catalyst weight have a positive effect on the reaction yield.
Fig. 3. Influence of each independent variable
on the reaction yield
Table 3. Regression variance analysis of the model
Source of variation | Coefficient | Sum of Squares | ν | Mean square | Fexp | Significance test |
Regression | – | 4816.8625 | 05 | 0963.3725 | 05224.36 | *** |
β0 | 76.5000 | – | 01 | – | – | – |
β1 | 10.8551 | 0942.6620 | 01 | 0942.6620 | 05112.83 | *** |
β2 | 20.8049 | 3462.7300 | 01 | 3462.7300 | 18781.21 | *** |
β11 | – 03.4375 | 0054.0175 | 01 | 0054.0175 | 00292.98 | *** |
β12 | 00.7500 | 0002.2500 | 01 | 0002.2500 | 00012.20 | ** |
β22 | – 09.4375 | 0407.1610 | 01 | 0407.1610 | 02208.37 | *** |
Residue | – | 0000.7375 | 04 | 0000.1844 | – | – |
Total | – | 4817.6000 | 09 | – | – | – |
*** : p ≤ 0,01 ; ** : p ≤ 0,025 ; * : p ≤ 0,05 ; NS : No significant; F0.01(5, 4)=15.52.
Figure 4 show the interaction between independent variables in three-dimensional surface plots.
Fig. 4. Response surface graphs for synthesis of anti-tuberculosis drug
The geometric representation of the reaction yield for this product according to the reaction time and the catalyst weight is shown in Figure 5.
Fig. 5. Contours of estimated response surface for synthesis
of anti-tuberculosis drug
Analysis of the contour plot shows that when the reaction time and the catalyst weight increase together or when the reaction time increases and the catalyst weight remaining unchanged and or when the catalyst weight increases and the reaction time remaining unchanged, then the reaction yield increases up to 95% in the experimental field. The main objective of this research is to determine the best reaction conditions for synthesis of (2E,6E)-2,6-bis(4-nitrobenzylidene)cyclohexanone. Then, using above-mentioned methodology for experimental design, the ranges of the parameters required to obtain optimum conditions were determined. In this optimization study, reaction yield was chosen as the objective function. Furthermore, optimum conditions are often calculated in the presence of some constraints, which ensure them to be more realistic. If the model used in the optimization study is an empirical one, high and low levels of the process parameters in the experimental design are considered, inevitably, as explicit constraints, in order to avoid extrapolation. Thus, the optimization problem for response is defined by equations 3 and 4:
Maximize: Y = high reaction yield (3)
−αi<Xi<+αi i=1,2 (4)
The investigation of contour plot (Figure 5) and the equation 2 showed that, if X1=1 and X2=0.75; the value predict from the results using response surface model is 95%. The experimental checking in this point, i.e. under the optimum reaction conditions such as: reaction time=5h and catalyst weight=550mg, with high reaction yield 96%, confirms this result. To obtain further evidence we studied the effects of different catalysts on the condensation reaction of 4-nitrobenzaldehyde with cyclohexanone. The results of this study are presented in Table 4.
Table 4. Comparative study for synthesis of product 3 using different catalysts
Entry | Catalyst | Reaction parameters | Yield (%) | References |
1 | InCl3.4H2O | 110°C/24h | 93% | [16] |
2 | KOH | EtOH/12h | 87% | [07] |
3 | DBSA | MeOH/H2O/Reflux/12h | 80% | [17] |
4 | KF/Al2O3 | MeOH/Reflux/Ultrasound/2h30min | 60% | [18] |
5 | Cu(OTf)2 | Solvent free/80°C/8h | 93% | [19] |
6 | I2 | CH2Cl2/9h30min | 89% | [20] |
7 | Na/FAP | H2O /Reflux/5h | 96% | Present work |
From table 4, it can be seen for example in the presence of InCl3 catalyst, it is necessary that this condensation should be heated to 110°C for 24 hours. In addition, we also note that the synthesis of product 3 catalyzed by P-Dodecylbenzenesulfonic acid DBSA under refluxing methanol–water mixture (1:1) for 12 hours give a yielding 80%. However, these methods have some drawbacks such as use of costly catalysts and toxic reagents and special catalyst preparation. Comparison of the results obtained by our catalytic processes with some of those reported shows the efficiency of this method because of shorter reaction times, high yields. The high reactivity and specificity of our Na/FAP coupled catalyst to their easy separation of the catalyst by simple filtration, which reduces the environmental problems makes it attractive alternatives to homogenous base reagents.
- 4. Conclusions
The optimization for synthesis of (2E,6E)-2,6-bis(4-nitrobenzylidene)cyclohexanone a drug against dormant version of the Mycobacterium tuberculosis H37Ra, catalyzed by modified Fluorapatite with sodium nitrate Na/FAP catalyst in aqueous media has been studied using central composite design. The model equation for the optimization of the reaction conditions for this synthesis was established. From this equation, it was possible to forecast the optimal reaction conditions for synthesis of this Anti-Tuberculosis Drug in high yield. This process bring advantages, such as high catalytic activity and easy separation of the catalyst by simple filtration, use of non-toxic and inexpensive catalysts and especially, elimination of salts and by-product pollutants. This new solid base catalyst becomes then a practical alternative to soluble bases.
References
- Rom WN, Garay SM, Tuberculosis, 2nd edition, Philadelphia, PA: Lippincott Williams & Wilkins, 2004.
- Bifani, S. Moghazeh, B. Shopsin, J. Driscoll, A. Ravikovitch, B. N. Kreiswirth, Molecular Characterization of Mycobacterium tuberculosis H37Rv/Ra: Variants: Distinguishing the Mycobacterial Laboratory Strain, Journal of Clinical Microbiology, 38 (2000), 3200-3204.
- Global tuberculosis report, 20th edition, World health organization, WHO/HTM/TB/2015.22, ISBN 978 92 4 156505 9, 192 pp., 2015.
- A guide to monitoring and evaluation for collaborative TB/HIV activities, World health organization, WHO/HTM/TB/2015.02, ISBN: 978 92 4 150827 8, 44 pp., 2015.
- Robert Stein, Bo-Young Jeon, Jeong-Yeon Kwak, Min-Kyong Song, Juan Pablo Patron, Sabine Jorg, Kyoungmin Roh, Sang-Nae Cho, and Stefan H.E. Kaufmann, Mutation in the Transcriptional Regulator PhoP Contributes to Avirulence of Mycobacterium tuberculosis H37Ra Strain. Cell Host & Microbe, 3 (2008), 97–103.
- Guidelines for treatment of tuberculosis, fourth edition, World Health Organization, WHO/HTM/TB/2009.420, ISBN: 9789241547833, 147 pp., 2010.
- Singh, J. Pandey, A. Yadav, V. Chaturvedi, S. Bhatnagar, A.N. Gaikwad, S.K. Sinha, A. Kumar, P.K. Shukla, R.P. Tripathi, A facile synthesis of ,’-(EE)-bis(benzylidene)-cycloalkanones and their antitubercular evaluations. European Journal of Medicinal Chemistry, 44 (2009) 1705-1709.
- Riadi, R. Mamouni, R. Azzalou, R. Boulahjar, Y. Abrouki, M. El Haddad, S. Routier, G. Guillaumet, S. Lazar, Animal bone meal as an efficient catalyst for crossed-aldol condensation, Tetrahedron Letters, 51 (2010) 6715-6717.
- Salehi, M. Dabiri, M.A. Zolfigol, M.B. Fard, Silica sulfuric acid as an efficient and reusable reagent for crossed-aldol condensation of ketones with aromatic aldehydes under solvent-free conditions, J. Braz. Chem. Soc., 15 (2004) 773-776.
- S. Abaee, M.M. Mojtahedi, S. Forghani, N.M. Ghandchi, M. Forouzani, R. Sharifi, B. Chaharnazm, A Green, inexpensive and efficient organocatalyzed procedure for aqueous aldol condensations, J. Braz. Chem. Soc., 20 (2009) 1895-1900.
- S. Abaee, M.M. Mojtahedi, R. Sharifi, M.M. Zahedi, H. Abbasi, K. Tabar- Heidar, Facile synthesis of bis(arylmethylidene)cycloalkanones mediated by lithium perchlorate under solvent-free conditions, Iran. J. Chem. Soc. 3 (2006) 293-296.
- Abrouki, M. Zahouily, A. Rayadh, B. Bahlaouan, S. Sebti, A natural phosphate and doped-catalyzed Michael addition of mercaptans to α,β-unsaturated carbonyl compounds, Tet. Lett., 43, (2002), pp. 8951-8953.
- Zahouily, Y. Abrouki, A. Rayadh, S. Sebti, H. Dhimane, M. David, Fluorapatite: efficient catalyst for the Michael addition, Tet. Lett., 44 (2003), 2463-2465.
- A Solhy, A Smahi, H El Badaoui, B Elaabar, A. Amoukal, A. Tikad, S. Sebti, D.J. Macquarrie, Efficient hydration of nitriles to amides catalysed by sodium nitrate modified fluorapatite, Tet. Lett., 44 (2003), 4031-4033.
- Abrouki, A. Anouzla, H. Loukili, R. Lotfi, A. Rayadh, M.A. Bahlaoui, S. Sebti, D. Zakarya, M. Zahouily, Central composite experimental design applied to the catalytic carbon-sulfur bond formation by fluorapatite catalyst, American Journal of Applied Chemistry, 1 (2013), 22-27.
- Deng, T. Ren, Indium Trichloride Catalyzes Aldol-Condensations of Aldehydes and Ketones, Synthetic Communications, 33 (2003) 2995-3001.
- Sheikhhosseini, S.A. Ahmadi, S. Sadeghi, P-Dodecylbenzenesulfonic acid (DBSA), a Brønsted acid-Surfactant Catalyst for Synthesis of α, α ‘-bis(substituted benzylidene)cycloalkanones with Electron-Withdrawing Substituent in Aqueous Media, Journal of Applied Chemical Research, 8 (2014) 25-30.
- T. Li, W.Z. Yang, G.F. Chen, T.S. Li, A Facile Synthesis of a,a0-bis(Substituted Benzylidene) Cycloalkanones Catalyzed by KF/Al2O3 Under Ultrasound Irradiatio, Synthetic Communications, 33 (2003) 2619-2625.
- Li, W. Su, N. Li, Copper Triflate-Catalyzed Cross-Aldol Condensation: A Facile Synthesis of a,a’-Bis(Substituted Benzylidene)Cycloalkanones, Synthetic Communications, 35 (2005) 3037-3043.
- Das, P. Thirupathi, I. Mahender, K.R. Reddy, Convenient and facile cross-Aldol condensation catalyzed by molecular iodine: An efficient synthesis of a,a’-bis(substituted-benzylidene)cycloalkanones, Journal of Molecular Catalysis A: Chemical, 247 (2006) 182-185.
About the Author : Prof. Dr. Kannan Vishwanatth.
Armed with Chemical Engineering degree, Dr Kannan Vishwanatth is currently the promoter Director of Hong Kong-based pharmaceutical company Rupus Global Limited.Dr Kannan Vishwanatth is a global opinion maker of contemporary issues & a much sought after speaker in various international forums. Dr Kannan is credited with reputation for innovation, social connections, track record for value creation and investor expectations for value creation. As a Research Scholar, Dr. Kannan has published many research papers & is associated with many top notch International Institutions as Editorial Reviewer. Dr. Kannan Vishwanatth is a global Citizen & a strong believer in Corporate Social Responsibilities. Over the years, Dr. Kannan has slowly transitioned away from Corporate World and into philanthropic & academic ventures. Dr. Kannan Vishwanatth, 45 years, is the Founder and Promoter & Managing Director of our Company. He holds a doctorate in Business Management (Ph.D.). He has an experience of 20 years in the pharmaceutical industry. As the Promoter & Managing Director, Dr. Vishwanatth, has been the backbone of our Company’s operations and is involved in formulating the Company’s strategy. Under his guidance, our Company, ventured into new geographies with a wide product range in various therapeutic segments. His vision and value system have guided the organization towards profitable sustainability. Believing in delegation of responsibility, Dr. Vishwanatth created a professional team and expects Rupus Global Limited to emerge as a global player across multiple therapeutic segments.
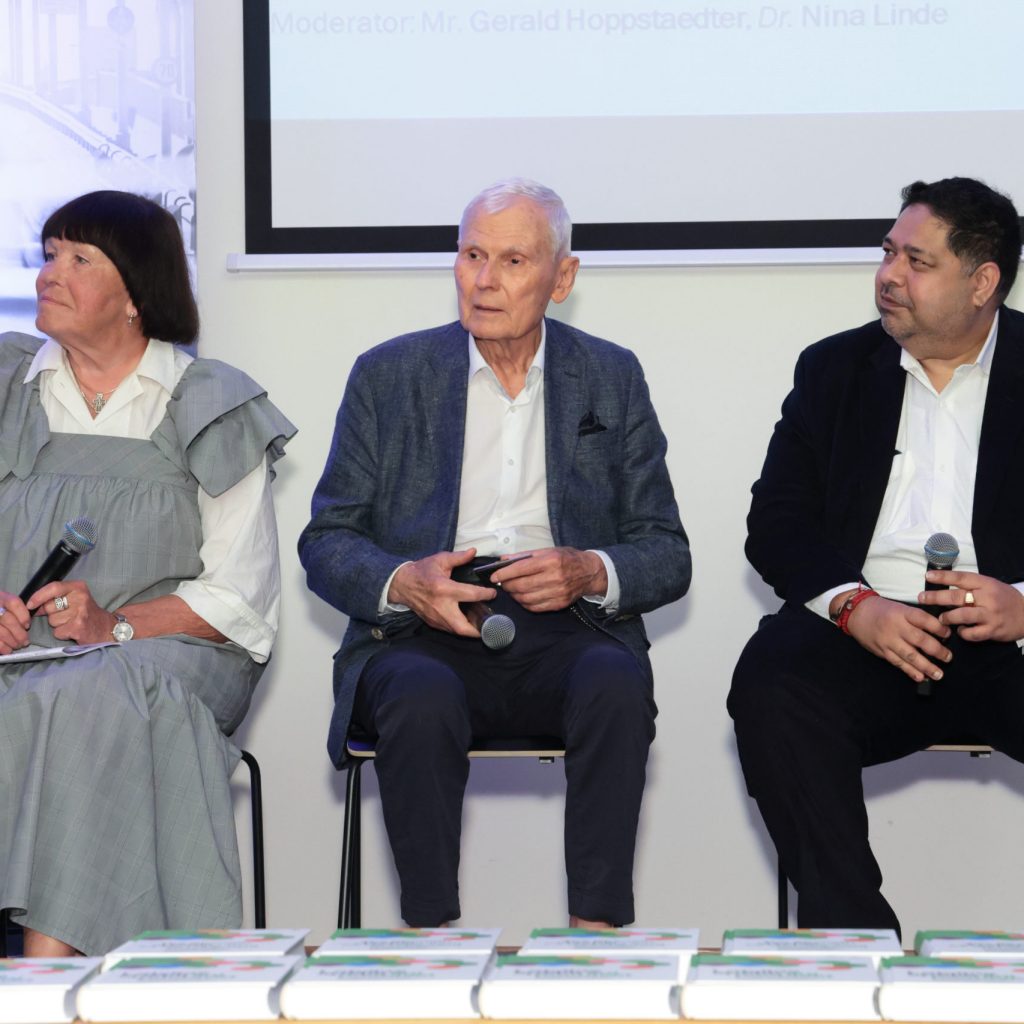